I just got back from the Granular Matter in Low Gravity conference in Erlangen, Germany hosted by the Friedrich-Alexander Universität. It was a fun week and it reminded me why I love working with granular materials in space.
Why Granular Matter is Important in Space
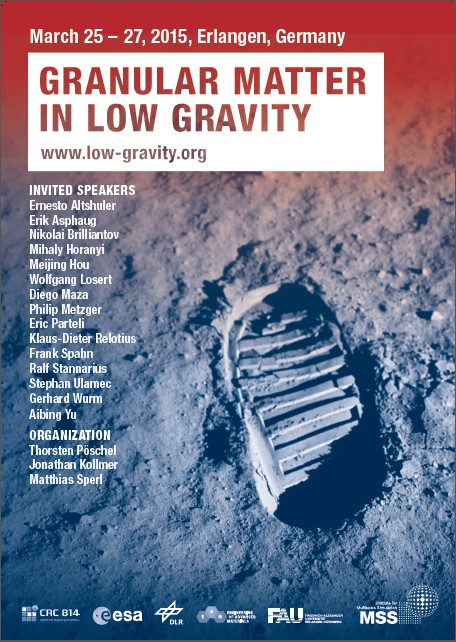
Granular matter is simply any material that is a collection of small bits, like beach sand or corn flakes. Physicists love it because it can act like a solid (example: you want walk on beach sand without sinking into it), or like a liquid (you can pour it through a funnel), or even like a granular gas. Surprisingly, we can’t predict how sand will behave from “first principles”, meaning that we can’t write fundamental equations of physics that will give us the right answers about sand. It’s one of the last areas of unsolved, everyday physics.
Now that’s quite a situation, because it turns out just about anywhere we can land a spacecraft will be covered with granular matter. That’s a result of the way planets form: a solar system has lots of leftover planet stuff in the form of asteroids, which constantly rain down, busting up a planet’s surface, turning its bedrock into the loose layers of broken-up material we call “regolith”. Regolith means “rock blanket”. It includes boulders, rocks, gravel, sand, and dust.
So regolith is a granular material, and it’s everywhere in space, and we have no fundamental equations to predict how it will behave. Nice.
It’s also (arguably) one of the two materials that humanity has the most experience working with here on the Earth. The other is…(wait for it)…water. We have been working with soil since forever — poking in it for potatoes, heaping it into shelters against the wind, digging wells in it to get water, crossing it on the backs of camels, irrigating it for our crops after we learned farming, and so on. And over the centuries we have developed many equations to help us predict how this ubiquitous granular material will behave. (The very first soil mechanics experiment was by none other than Charles-Augustin de Coulomb in 1776. The first in low gravity was by Buzz Aldrin in the Apollo 11 mission, 193 years later.) Nevertheless, our equations are all semi-empirical, meaning they are based on our experiences and not completely from first principles of physics. That’s a problem, because our experience with soil here on Earth doesn’t help very much when we go to other planets where gravity is less, the atmosphere thinner, the soil less weathered, and so forth. Granular matter behaves differently depending on what environment you put it into. That’s why we need equations from first principles and not just from our experience on Earth.

I have done many experiments with sand and with simulated lunar and Martian soil in the reduced gravity aircraft (what people still call “the Vomit Comet”, even though it’s not the same aircraft). In low gravity, sand starts to act more clingy, like powdered confectioner’s sugar. It’s remarkable the first time you see that happen. For example, we had a container of simulated lunar soil, and in Earth’s gravity you could tilt it and the soil would slide down into a heap just like regular sand. But in lunar gravity in the aircraft, you could turn the container completely upside down and the soil would hang in the top of the container. You had to shake the container to get it to fall down, and when it did it would first form cracks like a fracturing solid, and then it would bust apart, fall, and splash around the bottom of the container like agitated, black milk. ‘Twas amazing to behold.
So here’s the situation: granular matter is one of the two materials we’ve used the most in this world, and yet we can’t predict it’s behavior outside this world, and it is everywhere we want to go. And on top of that, it’s where most of the resources in space are located, so we need to work with granular matter more than any other substance in space.
What We Must Do with Granular Matter
When we go to another world in space, we must:

- Land on granular matter with rockets
- Drive on it
- Dig in it
- Process it for resources
- Build with it as a construction material, and
- Study it for science
When I was at NASA, this is what I spent all my time working on. We were developing technologies to do all of these things with granular matter. (That’s why the lab was called the Granular Mechanics and Regolith Operations Laboratory.) I’m still working on it now at the university, and so are my colleagues in the Swamp Works and elsewhere in NASA, along with many other researchers in academia and business.
Now it’s one thing to develop a technology intended to work with regolith on another world. It’s another thing to prove that it will really work after the technology arrives there. I’ve seen technologies that worked great in Earth’s gravity that didn’t work at all when you put them into the reduced gravity aircraft. And there are some technologies that are simply too big or too slow to test inside an airplane, which provides only 20 seconds of low gravity at a time. And some technologies require hard vacuum as well as low gravity, but sadly we can’t fit better than a tiny vacuum chamber inside the airplane. So how do we test the interaction of technology with granular matter as it will behave in the full glory of the space environment?
I think the best strategy is to develop simulation algorithms that will predict how granular matter behaves on other planets. Then, we can set the environment in our virtual world to anything we like. We can set it to the conditions of the reduced gravity aircraft, or the lab, or the field test on top of a volcano, or to the distant planet itself. Then, we can compare how the technology fared in the reduced gravity aircraft and all the other test locations against how the software predicted it would fare. If the software predicted the behavior correctly in each case, then we can feel more confident that it predicts the behavior on the planet correctly, as well. Thus, the simulations allow us to cobble together a test program using whatever inadequate test conditions we can afford, in airplanes or on volcanoes or in the lab, and despite the inadequacy give us some real confidence before we fly the mission.
Now, this strategy only works if the simulation software is good. But remember, we don’t have any fundamental equations of granular material behavior. We have made lots of progress, but we have lots of work still to do. So this is why we have communities of people trying to solve fundamental granular physics. When we have been forced to build things without adequate software, we sometimes have hardware failures in space. You might remember that Mars rover Spirit became stuck in sand, as did the Chinese lunar rover Yutu. And when Curiosity landed on Mars, the Sky Crane’s thrusters blew gravel onto the rover and that apparently broke one of its two wind sensors. I could go on and on telling of problems with granular materials defeating our space technology. These kinds of problems could prove fatal if we have a mission with humans on a faraway world, and that makes it much more important.
So we had a great conference this past week in Erlangen. We talked about 3D printing entire buildings out of lunar soil, how sand dunes migrate on Saturn’s moon Titan, how planets form by grains colliding in space, and why Brazil nuts rise to the top of a mixed nut container when shaken. (Hey, it’s important to know if you want to predict how granular materials will behave!) It was great seeing some colleagues whom I have not seen in many years. We made some plans for collaboration. I hope to fill you in on some of the progress we are making, soon.
(For now, if you want to see some of my prior work on granular physics theory, check out this paper here, which was published here. Or if you want to see some experimental work, check out this one.)